Creating an X-ray Nebula in the Laboratory: Spectral
Diagnostics of Neon Photoionization
Experiments on the Z-Machine
D. S. Conners, D. H. Cohen (Swarthmore College &
Prism Computational Sciences, J. J. MacFarlane (Prism Computational Sciences),
D. A. Liedahl (Livermore
National Laboratory), J. E. Bailey (Sandia National Laboratory)
Gas Cell Experiments
Experimental
Design
VisRad Viewfactor Simulations of the X-ray Source
Motivation
Spectral
Diagnostics
Backlit
Absorption
Spectral
Diagnostics
Emission









Introduction:
We have begun a campaign of ride-along shots on the Z-machine at
Sandia in order to study the response of low-density gases to
irradiance by strong x-rays. Our
interest is in the excitation/ionization kinematics and the
physical conditions of the x-ray photoionized plasma. We would like to minimize gradients, shocks,
and collisional effects and isolate the atomic physics. The motivation behind these
experiments is to study basic physics, explore fusion and X-ray laser
applications, and to benchmark codes used to study
astrophysical plasmas.
The experimental package consists
of a cm-scale neon-filled cell with mylar windows, mounted
several cm from the Z-machine’s current return can,
inside of which is the pinch itself.
Ultimately, we plan on making simultaneous,
time-resolved absorption and emission spectroscopic
measurements of the gas cell, which can be filled with
a variety of gases, including mixtures. The absorption
spectroscopy uses the pinch itself as a backlighter.
Experiments already completed
have used 30 Torr neon (nion ~ 1018 cm-3) observed in absorption
with a time-integrated spectrometer.
With the advent of
high-resolution (l/Dl ~ 1000) x-ray
spectroscopy in the last several years, a wealth of
spectroscopic data for photoionized sources has been generated, and
the level of detail and accuracy demanded of models has increased
dramatically.
By producing and measuring a
well-characterized x-ray photoionized plasma in the laboratory,
we hope to benchmark the codes used to model x-ray spectra
of active galactic nuclei, x-ray binaries, and other astrophysical sources
for which photoionization dominates collisional ionization.
The Chandra telescope (left) and XMM telescope, both launched in 1999, are the first high-resolution spectroscopic missions for the astronomical community.
Both employ gratings. X-ray missions planned for the future will use
microcalorimeters for high spectral
resolution.
The conditions in the plasma in proximity to the compact object
are traditionally described by the
ionization parameter (flux/density), which is a
measure of the dominance of radiation processes over collisional
processes. Contours
of constant ionization parameter (logarithmic, in cgs units) are shown
for the HMXRB Vela X-1 (above, right; Figure from Sako et al. Ap.J., 525,
921 (1999)). Our previous experiments
have achieved an ionization parameter of ~7, but significantly
higher values are possible.
Viewfactor simulations of the pinch and target are used
to calculate the incident
spectrum on the gas cell, and also to investigate the spatial uniformity of the irradiance on
the cell.
Accretion onto compact objects leads to photoionization-dominated X-ray spectra
The circumstellar gas near the
source of hard x-rays is highly ionized--overionized for
its temperature compared to coronal equilibrium--and produces an x-ray spectrum
dominated by radiative recombination continua and recombination cascades.
Calculation of iron emission spectrum for a photoionized plasma
(left) and a collisional plasma (right). Though the ionization distribution is the
same in both models, the spectra are quite different (and
the photoionized plasma is much colder). (Figure taken from Liedahl et al. Ap.J.,
350, L37 (1990).)
Two snapshots (an early time, during the low-temperature foot of
the pulse on the left and a late time from the high-temperature peak)
from a VisRad simulation of the imploding pinch and gas
cell. The four frames, in each snapshot
are a complete view of the pinch, current return can, and target
(represented by the flat square) (top left), a zoomed-in view
of the pinch through the current return can (the opaque slats
of which are represented by ‘scaffolding’ in the front so as not to obstruct
the view in this representation) (top right), a view from inside the
can, looking through the slats at the target (lower left),
and the instantaneous spectrum incident on the front of the gas
cell (lower right).
Viewfactor simulation output is
used as input to our hydrodynamic
simulations.
The time-dependent pinch drive temperature (left). On the right, we show, for
one simulation time, the incident spectrum (red) and blackbody equivalent
(black) for one point on the front of the cell. Note that the incident spectrum is
quite non-Planckian.
Temperature (left) and density
(right) at four different times in the simulation of our experiments. The
radiation is incident from the left.
The initial neon gas density is 1018 cm-3. Note the shock waves launched from the mylar walls at late times. Note also the radiation wave (as evidenced
by the temperature
gradient) traversing the gas at t=100 ns.
Conclusions
from hydro: To optimize uniformity of the physical conditions,
a lower gas density is desirable. To
minimize compression of the gas by the mylar walls, thicker
walls are desirable. By doping the
walls or coating them with thin foils, we may be able to optimally filter
the x-rays flowing into the gas cell as well as putting off the implosion
of the gas by the wall plasma until very late times.


A series of Ka absorption spectra, representing three different
sets of physical conditions in the gas cell (warm
to hot from top to bottom). Spectral resolution is 5 eV
(FWHM).
The time-integrated absorption spectrum from
our first round of shots (top, above) are well-matched by our
calculations (lower, above).
A section of our raw data from the same shot (above).
Note the very high principle quantum number lines
of He-like neon.

A spectral simulation of neon, based
on a representative hydro calculation.
The intrinsic spectrum
(left) shows numerous lines of B-like through H-like neon, as well as a
recombination edge (near
1200 eV) and recombination and free-free continua. At 2 eV (center) and 5 eV (right) spectral resolution, many of the features are
blended. The 2 eV resolution spectrum
is probably good enough to
quantitatively test atomic/spectral models, whereas a lot of information is lost at a resolution of 5 eV.
Sandia’s Z-Machine is the worlds most powerful source of X-rays
An pulsed-power imploding
tungsten wire array: creating a cm-scale, >150 eV plasma,
generating 200 TW of X-rays in a ~10 ns pulse (2 MJ of X-rays).
The
Z-Machine’s Marx capacitor banks before a ‘shot’.
Experimental Schematic
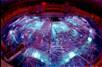



Experimental
Design
Hydrodynamic
Simulations of the Gas Cell
Data

Conclusions
•Absorption spectra can be
measured in this configuration;
•Ionization parameters of ~100
should be achievable at nion~1017 cm-3;
•Measurement of recombination
spectra and accurate wavelengths of inner-shell transitions
should be possible in the next round of experiments.